Wi-Fi Unplugged: The Radio Science Behind Wireless Internet
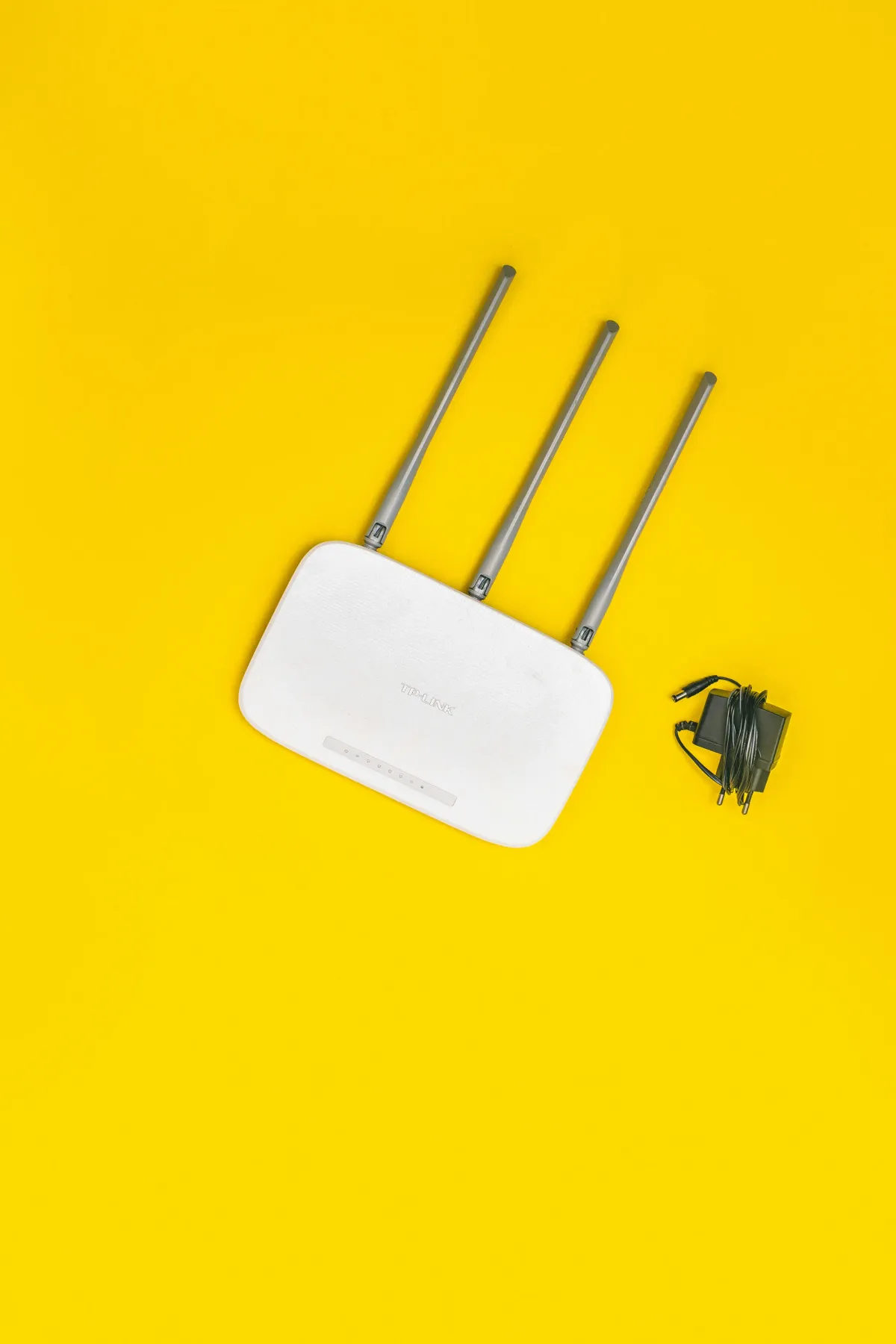
Welcome to the first installment of our Wi-Fi technical series. This post will explore the fundamental principles of radio wave transmission, modulation techniques, and the mechanisms that enable Wi-Fi to transmit data through free space. While Wi-Fi is an everyday convenience, a deeper understanding of its foundations can provide insight into both its capabilities and limitations, as well as improve troubleshooting approaches. Wireless networking is not magic—it is the result of decades of advancements in electromagnetic theory, signal processing, and engineering innovations.
The Role of Radio Waves in Wi-Fi Communication
At its essence, Wi-Fi is a form of radio communication. It relies on electromagnetic waves to transmit data, much like AM/FM radio, television broadcasts, and mobile networks. However, instead of carrying analog audio or video signals, Wi-Fi encodes and transmits digital data packets. These packets travel invisibly through the air, allowing seamless device communication without physical connections.
Transmission and Reception Mechanics
A Wi-Fi router functions as a transmitter, converting digital data into an electromagnetic signal that propagates through space. A client device, such as a laptop or smartphone, acts as the receiver, detecting and decoding this signal back into usable data.
- The router generates an electrical signal, which is fed into an antenna.
- The antenna converts this signal into an electromagnetic wave, which propagates outward in all directions or in a specific beam.
- The receiving device’s antenna captures the wave and converts it back into an electrical signal.
- The device’s radio circuitry deciphers the encoded data and processes it accordingly.
This process occurs at extremely high speeds, with millions of wave cycles per second, allowing for near-instantaneous data exchange. The effectiveness of this transmission depends on signal strength, environmental interference, and the efficiency of encoding techniques.
Understanding Radio Waves
At its core, a radio wave is an oscillating electromagnetic signal that can be described mathematically as:
In this equation, A represents the amplitude, or the wave’s strength, ω (omega) is the angular frequency, which determines how fast the wave oscillates, and φ (phi) is the phase, dictating the wave’s position in time. These three properties—amplitude, frequency, and phase—define the characteristics of a wave and, crucially, serve as the foundation for encoding information in wireless communication.
Carrier Waves and Signal Encoding
Radio communication relies on carrier waves to transport encoded information. A carrier wave, by itself, is just a steady, unmodulated signal—essentially a blank canvas. To make it useful, data must be encoded onto it through a process called modulation, where the wave’s frequency, amplitude, or phase is adjusted to represent information.
Wi-Fi operates in the 2.4 GHz, 5 GHz, and 6 GHz frequency bands, but these frequencies alone don’t tell the full story. Within each band, the available spectrum is divided into channels—smaller frequency ranges that allow multiple networks to operate simultaneously without interfering with each other.
What Are Wi-Fi Channels?
Imagine a multi-lane highway. The entire highway represents a frequency band, like 2.4 GHz or 5 GHz. Each lane, however, is a separate channel, allowing different streams of data to travel independently. If too many cars (or Wi-Fi networks) pile into the same lane, congestion occurs, slowing everyone down. But if traffic is spread across multiple lanes, everything flows more smoothly.
In the 2.4 GHz band, there are only 14 channels, and because each one is relatively wide, they overlap with one another. This overlap leads to interference, especially in areas with many Wi-Fi networks. To avoid congestion, only three channels (1, 6, and 11) are typically used, further limiting capacity.
The 5 GHz band improves on this by offering a much larger number of non-overlapping channels, reducing interference and improving overall performance. This is why modern Wi-Fi routers often prioritize 5 GHz for high-speed connections.
The 6 GHz band, introduced with Wi-Fi 6E, expands this even further by adding even more channels—with no overlap at all. This means devices can communicate with much less interference, making 6 GHz the best option for ultra-fast, high-performance wireless connections in areas where congestion is an issue.
Why These Frequencies?
To understand why Wi-Fi uses these specific frequency bands, we have to look at how radio waves behave. Lower frequencies, like 2.4 GHz, travel farther and penetrate walls more effectively, making them ideal for whole-home coverage. However, they are also more susceptible to interference, since many household devices—such as Bluetooth devices, cordless phones, and microwave ovens—emit signals in this range.
Higher frequencies, like 5 GHz, don’t travel as far but can carry more data. Since this band offers more channels and less interference from non-Wi-Fi devices, it became the preferred choice for faster, high-bandwidth activities like streaming and gaming.
The 6 GHz band takes this even further by providing an entirely new set of channels with minimal interference. The tradeoff, however, is that the signal has an even shorter range and struggles to penetrate walls, making it best suited for open spaces or close-range, high-speed connections.
Beyond physics, there’s also a regulatory reason for these choices. Wi-Fi operates in what’s known as the ISM (Industrial, Scientific, and Medical) bands, which were originally designated for non-commercial uses like microwave ovens and medical equipment. Since these bands were unlicensed and freely available, they became a natural fit for wireless communication technologies like Wi-Fi. Over time, as demand for faster and more reliable internet grew, regulators expanded the available spectrum, leading to the multi-band Wi-Fi systems we use today.
Ultimately, the selection of 2.4 GHz, 5 GHz, and 6 GHz represents a balance between range, speed, and interference. Lower frequencies provide broad coverage, while higher ones enable faster data transfer with less congestion. By intelligently switching between these bands and their available channels, modern Wi-Fi devices ensure users get the best possible connection for different environments and applications.
Modulation: Encoding Data onto Radio Waves
Simply transmitting a raw radio wave isn’t enough to carry information—Wi-Fi devices need a way to encode digital data onto these waves. This is where modulation comes in. Modulation is the process of altering a carrier wave so that it represents meaningful data, much like varying the pitch and tone of a voice to convey different words and emotions.
There are several ways to achieve this. Amplitude Modulation (AM) changes the wave’s strength, making it louder or softer, while Frequency Modulation (FM) shifts how fast the wave oscillates. Another method, Phase Modulation (PM), tweaks the timing of the wave’s peaks and valleys to encode information.
Wi-Fi, however, needs to pack as much data as possible into each transmission, so it uses a more advanced technique called Quadrature Amplitude Modulation (QAM). QAM is a hybrid approach that modifies both amplitude and phase simultaneously, allowing each wave to carry multiple bits of data at once. This is what makes high-speed wireless connections possible—by squeezing more information into each symbol, Wi-Fi can transmit data at gigabit speeds while using the available radio spectrum as efficiently as possible.
QAM: The High-Capacity Encoding Method for Wi-Fi
QAM enables Wi-Fi to transmit multiple bits per symbol by adjusting amplitude and phase variations. Some widely used QAM schemes in Wi-Fi standards include:
- 16-QAM: Represents 4 bits per symbol.
- 64-QAM: Represents 6 bits per symbol.
- 256-QAM: Represents 8 bits per symbol (utilized in Wi-Fi 5 and Wi-Fi 6).
- 1024-QAM: Represents 10 bits per symbol (used in Wi-Fi 6 and beyond).
Higher QAM orders allow for increased data transmission rates but require stronger signal integrity. As signal strength diminishes due to distance, interference, or obstacles, the network may dynamically shift to a lower QAM level to maintain stability. This adaptability ensures a balance between speed and reliability.
Practical Implications of Wireless Transmission
A solid grasp of radio transmission principles aids in diagnosing and resolving common Wi-Fi issues:
- Interference: Competing radio sources, such as Bluetooth devices, cordless phones, and microwave ovens, can disrupt signal clarity. The presence of other Wi-Fi networks on the same or overlapping channels can also lead to congestion and performance degradation.
- Signal Attenuation: Increased distance from the router weakens the received signal, reducing achievable data rates. Physical obstructions such as walls, furniture, and electronic appliances can absorb or scatter Wi-Fi signals, further reducing range and effectiveness.
- Multipath Propagation: In indoor environments, signals can bounce off surfaces, creating multiple copies of the same signal arriving at different times. Modern Wi-Fi devices use technologies like MIMO (Multiple Input, Multiple Output) to mitigate these effects and improve throughput.
- Bandwidth and Channel Selection: Different frequency bands offer varying levels of performance and interference resistance. Proper channel selection can significantly enhance network reliability, especially in congested areas.
As Wi-Fi technology advances, understanding these concepts becomes increasingly relevant for optimizing network performance. In the next installment of this series, we will delve into Wi-Fi channels and frequency bands, explaining how networks mitigate interference and optimize throughput.
Stay tuned for a deeper exploration of wireless networking!